
RESEARCH
EVOLUTION OF NEURAL CIRCUITS
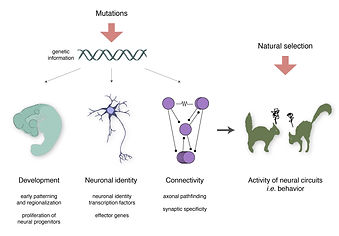
Our single-cell RNAseq approach revealed that simple cortices are a mosaic of evolutionary conserved and of new types of neurons. Do ancient neuronal types retain the same "functions"?
We are exploring this using calcium imaging, behavioral experiments, and viral tools in amphibians. With this work, we want to learn in general how the molecular evolution of neuronal types relates to the evolution of the neural circuits to which they belong.
MECHANISMS OF NEURONAL DIVERSIFICATION
Neurons are the building blocks of the brain. Neuronal types change over evolutionary time. We study how neuronal identities evolve in the vertebrate brain.
Our model system is the dorsal telencephalon or pallium, which is the most diverse area of the vertebrate brain. This area includes the mammalian six-layered cortex, and simpler structures in non-mammals. We want to understand how the diversity of neuronal types and tissue architectures is generated in the vertebrate pallium, starting from similar progenitor cells.
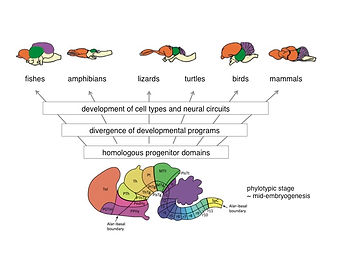